It is particularly poignant, now that we are in the middle of the covid crisis (that also stopped this blog), to reflect on what happens after the catastrophe. We have no idea of what we world we live will be, only that it will be different, that we will miss the past, that we will see something new unfold.
The same holds for mass extinctions. I’m working on a feature on extinction recovery, something I curiously forgot discussing when writing my book, and so I will drop some reading notes here. As it often happens, one misses the best papers right after publishing something, and I don’t know how I managed to miss the brilliant review by Pincelli Hull on life in the aftermath of mass extinctions. I mean, just the sentence “Rather than a plodding tortoise, extinction is a hare – racing in fits and starts” would have been perfect in my book. Oh well, it’s never too late.
The first point of Hull is that extinction recovery and species radiation takes place in a strange world. The world right after Chicxulub or after the Permian is not business as usual, with just some players missing. It is a bizarro world of precarious ecosystems with entire parts missing, of still recovering geochemical cycles. Disaster taxa dominate, entire ranges of body size are gone, the atmosphere is still recovers. It is in that arena that the destinies of evolutionary lineages are played. Take it after the Permian catastrophe:
Coral and metazoan reef systems were replaced by microbial carbonate mounds for up to six million years [16]. Key marine functional types including macroalgae, metazoan suspension feeders, mobile predators and deposit feeders were lost or rare for at least the first million years [16]. Complex burrowing of benthic sediments remained relatively rare for yet another ~4 million years […] In the aftermath of the PT extinction, certain
features were preserved in rocks that had not occurred since
abundant metazoans fully colonized the soft sediments of the
seafloor [55]. These features, and other anachronistic structures
[56], are important because they imply that certain ecological
strategies were so rare (or even absent) that they no longer
had a readily observable effect on ecosystems.
Something that comes up from different lines of evidence is that recovery is never a monolith: different environments and groups recover at different speed:
Although ammonites suffered very high levels
of extinction during the end-Permian, they diversified within
the first 2 million years after the extinction […] Benthic taxa, by contrast, generally took more than 5 million years to fully recover pre-extinction levels of diversity and community complexity […] Regardless of the cause, variation in diversification rates amongst taxa is typical of mass extinction aftermaths more generally. On land, some ecological strategies lost at the extinction boundary re evolved immediately while others, like small-bodied insectivory and large bodied herbivory, took more than 15 million years to reappear [64], resulting in distinct Early Triassic food-web structures in the meantime [65].
However there is an act of balance between the intrinsic factor, such as different competition or niches opening and closing, and instead extrinsic factors such as the quenching of volcanism after the main extinction event (Hull reminds that volcanic outgassing and anoxia were still present for millions of years in the Triassic), or the carbon cycle stabilization. Ammonite diversification in the Triassic seems coupled to the carbon cycle, for example. Post-extinction ecosystems seem weirdly unstable; for example various dynasties of dominance and decline follow each other for coccolithophores in the Paleocene, with no apparent reason.
Hull here posits a theory, that these two factors cannot be taken in isolation. Geochemistry affects ecosystems but ecosystems affect geochemistry as well:
On a global scale, the structure and function of ecosystems affects key earth-system processes, such as rates of weathering, soil formation, organic carbon sequestration, nutrient availability and recycling, and the availability of key substrates such as soils and reefs [78, 79, 80]. These processes move various elements between earth system reservoirs, for instance from the ocean to the atmosphere or from soils to streams. On a global scale, the time it takes for various elements to move, on average, between reservoirs can be quite long (100 years to 10 million years) [81]. As various earth-system reservoirs and fluxes change, they have the scope to, in turn, affect ecosystems on a global scale, because they alter the prevailing nutrient availability and environmental conditions
This reciprocal feedback results in what Hull calls earth system successions: not only biomes are different after mass extinctions, but the whole functioning of the planet. The extinction is thus followed by a state of instability while the systems comes back to a novel equilibrium. The waves of disaster ripple for millions of years before settling down.
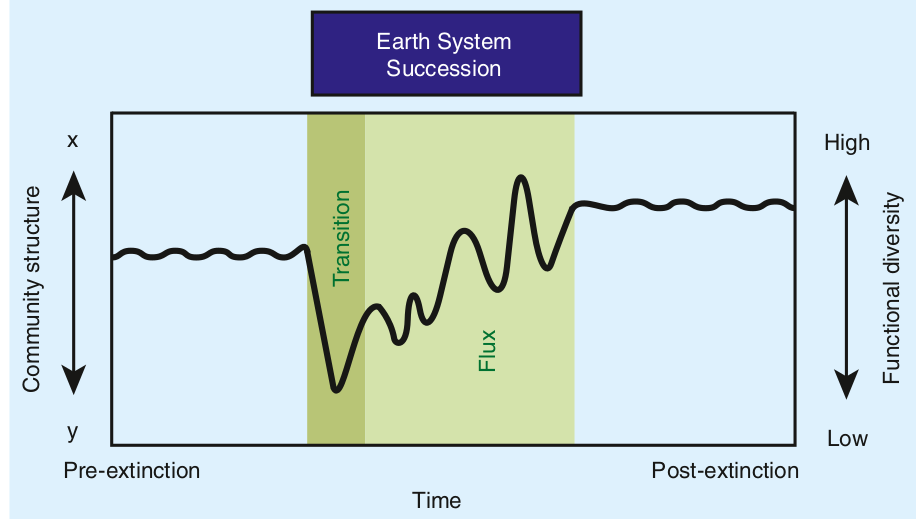
Hull also reminds us that mass extinctions are not the only game in town when it comes to major evolutionary upheavals. The Paleocene-Eocene warming event was a minor extinction event but a major turnover in megafauna (modern whales and pinnipeds arise there). The rise of flower plants during the Cretaceous was a massive evolutionary event in the history of Earth but it did not follow any major extinction, as far as we know.
What Hull teaches us is that mass extinctions are not point events; they are ripples of enduring change that interweave with other ever changing conditions:
In the prolonged aftermath, ecosystem change across the globe exerts an evolutionary influence distinct from the extinction itself, with a timing characteristic of the earth system (i.e., earth system succession). As such, mass extinctions should not be considered as macroevolutionary point events, but rather as prolonged intervals of varying selection spanning the mass death and subsequent radiation of taxa.
A deep, sobering perspective. And I want also to note: I believe such insight can come only from the awe and respect Hull has for the monumental task of reconstructing Earth’s history, something that we should all consider. Speaking of the Permian:
“To step back for a moment, just consider how remarkable
this hypothesis is — to argue that we can trace the trigger of
mass extinction a quarter of billion years ago to a single pulse
of volcanism in Siberia and its cascade of environmental effects.
It is astounding and relies on dramatic improvements in our
ability to precisely date the geological time scale, to discern
the relative amount of time captured in very thin layers of rock
and to measure various aspects of the environment with
geochemistry.”
It is marvellous to live in the age of humanity when this history unfolds under our eyes. To see such a majestic landscape in time and be moved by it, is what makes it possible for us to appreciate its complexity.