I have a fondness for parasites. Not on my body, of course, but as biological entities go, they’re among the most remarkable examples of specialization, adaptation and bodyplan plasticity. Parasites can go very far in becoming unrecognizable members of their own group, as Sacculina or pentastomids demonstrate… but I digress.
Parasites are also useful to understand life history and evolution. A nice factoid is that we have an idea of when humans started to use clothes thanks to lice. Body lice need clothes to survive (that’s where they nest), and we know they split from head lice 170.000-83.000 years ago. Thus probably clothing can’t be younger than that.
Now, what happens to parasites when their host goes extinct? They tend to go extinct as well, since most parasites are incredibly specific. This is a hidden biodiversity loss that we tend to overlook, but with each extinction of a free living species we lose also the whole parasitic ecosystem focused on that species -from viruses and bacteria to bugs and worms. Species do rarely die alone.
Viceversa, what happens to parasites after a mass extinction, when survivors start to diversify, often explosively, and reoccupy an empty world? A clue comes from a paper published on Communications Biology by Robert de Moya et al. that examines the diversification of bird feather lice after the K/T event. The answer is: they move around a lot.
The three groups of living birds -paleognaths (ostrichs, kiwis, tinamous, moa…) , Galloanserae (hen and ducks) and Neoaves (everything else)- diverged before the K/T event. We know that because we know at least a member of Galloanserae, the duck-ish bird Vegavis iaai, from before the end of the Cretaceous. If lice followed loyally their hosts, we should expect that they would have diversified also before K/T. Paleognath-loving lice on paleognaths, galloanserae-loving lice on ducks and hens, and so on.
But it is not so. de Moya et al. show genomic evidence that bird feather lice diversified about 50 millions of years ago, after the end-Cretaceous mass extinction:
Using the louse phylogenomic data set, we also conducted a dating analysis using calibration points external to the clade of feather lice. These dating analyses indicate that feather lice began radiating around 50 Mya, somewhat after the K-Pg boundary (Fig. 2), which is similar to previously published studies4. Thus, feather lice began to diversify following the origin of most modern avian orders1,2. […] Rather, overall the cophylogenetic analyses suggested that multiple host-switches have taken place by lice among modern groups of birds. The results of Jane7 cophylogenetic analyses indicated that the ancestral host of feather lice was the common ancestor of the Galloanserae
So, all living birds must thank ducks, hens and their relatives if they suffer feather lice. The ancestors of modern paleognats and Neoaves must have had their own lice, but they disappeared for some reason after the K/T event. The jumps were multiple and complex, apparently:
Cophylogenetic analyses suggest host-switching occurred from other birds to palaeognaths at least three times (Supplementary Tables 1 and 2, Supplementary Fig. 2) depending on the host tree evaluated (three host-switches in comparison with the Jarvis et al. tree1 and four host-switches in comparison with the Prum et al. tree2). All analyses indicate one of these host-switches to Palaeognathae was from an ancestor within Galloanserae to the ancestor of emus. Furthermore, two consistent host-switches to palaeognaths originated from the ancestors of frogmouths and potoos, two early diverging lineages of Neoaves.
Apparently, jumps between hosts were favoured by a common environment -not surprising, but nice to see that popping out of the analysis:
For example, both of the recent avian phylogenomic studies recovered a large group (Aequorlitornithes) of water associated birds1,2. We also found that the feather lice of these birds tended to be closely related. However, feather lice from some other birds associated with water, such as ducks and cranes, also fall within this clade of lice. Cophylogenetic reconstruction (Supplementary Fig. 2) suggests that ducks and cranes acquired their feather lice through host-switching from an ancestral flamingo8 (a member of the Aequorlitornithes). Thus, host-switching in these cases might have been facilitated by a shared aquatic habitat. We also reconstructed the acquisition of feather lice from two main lineages of predatory birds, hawks and falcons, as being the result of host-switching from other avian lineages (Supplementary Fig. 2). Hawks, for example, sometimes acquire feather lice from their prey9. Thus, predation on other birds may facilitate host-switching by feather lice to raptors10.
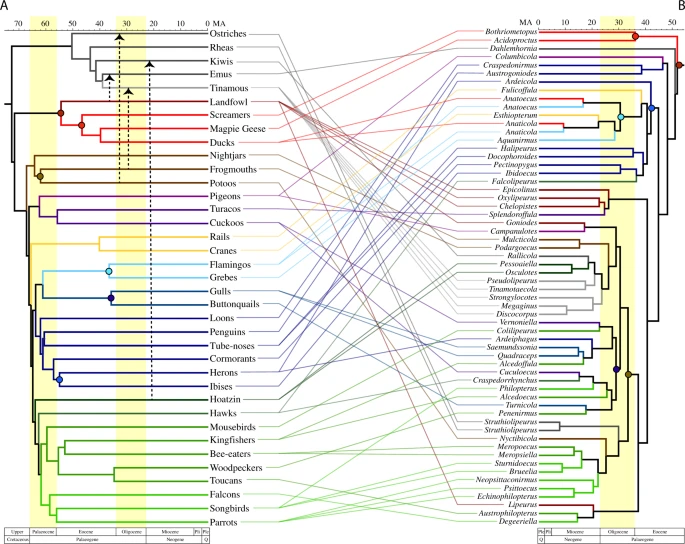
It seems that feather lice have an advantage on mammalian lice: they do not elicit an immune response from their host, which is probably a significant factor that makes it hard for them to change hosts (you have to re-adapt to a new host that will mount a significant immune response to you). But they also note an intriguing coincidence:
Numerous accounts have documented the ability of feather lice to attach to winged hippoboscid flies (louse flies, Diptera) and disperse between hosts via phoretic hitch-hiking11,12. The divergence of avian feeding hippoboscid flies is estimated to have occurred up to around 52 Mya13 providing opportunities for ancient host-switching via phoresis.
Whoa. Parasitic bugs acting as carriers of other parasitic bugs, this I did not know. And these carriers evolved roughly when the feather lice started to disperse. I wouldn’t bet on this being the reason all this host shuffling mess happened, but it surely it makes me scratch my feath…erm, head.
In summary, it is interesting to see how the diversification of birds and their parasites was all but monotonous. Birds diversified, lice of a lineage started jumping around and moved the other lice away (again, an extinction of parasites we will never know much about), perhaps the emergence of other parasites helping around. Birds, like any other being, are actually environments hosting an ecosystem, and species can move between these environments, forget their previous environment and evolve to occupy a new one.
After all, what loyalty can you expect from parasites?
The paper is: Moya, R.S., Allen, J.M., Sweet, A.D. et al. Extensive host-switching of avian feather lice following the Cretaceous-Paleogene mass extinction event. Commun Biol 2, 445 (2019) doi:10.1038/s42003-019-0689-7